When atoms are irradiated with light, they react within femtoseconds. In order to observe such fast-moving reactions in detail, an experimental setup is needed that works on equally small time scales, otherwise the images become "blurred". At the X-ray laser European XFEL (short for "X-Ray Free-Electron Laser") near Hamburg, researchers have set themselves the goal of developing just such an experimental setup and using it to penetrate the atomic depths of chemical reaction dynamics. With great success: In April 2022, they once again made headlines in the press when a EuXFEL research team succeeded in achieving a record time resolution of about 15 femtoseconds, the best resolution ever achieved in a so-called pump-probe experiment at an X-ray free-electron laser.
Such time resolutions allow for completely new insights into the dynamics of ultrafast processes, for example the temporal change of biomolecules in the course of a drug intervention. Thanks to the ultrashort light flashes of the European XFEL, processes that are much too fast for the human eye can be captured in sharp femtosecond snapshots and then assembled into a movie – as if one were following the individual steps of a chemical process in slow motion at the atomic level! This raises hopes, for example, of new insights into the efficient splitting of water in plants and thus optimized photosynthesis on an industrial scale.
The European XFEL also opens up completely new perspectives for information technology. For example, with the help of a special apparatus, the X-ray laser generates circularly polarized light, which could be used to erase and rewrite the magnetization of data carriers ultrafast in the future.
Last but not least, the experiments at the European XFEL pave the way for the great dream of structural biology: the structure determination of single, non-crystallized molecules. Conventional investigation methods require that the molecules be shock-frozen or crystallized beforehand. But the light from the X-ray laser is so bright that even molecules of which only very small crystals can be grown (membrane proteins, for example) can be studied. In the future, the researchers hope it will also be possible to study individual molecules so that crystals can be dispensed with altogether. In this way, a three-dimensional mapping of the nanocosmos is coming closer and closer!
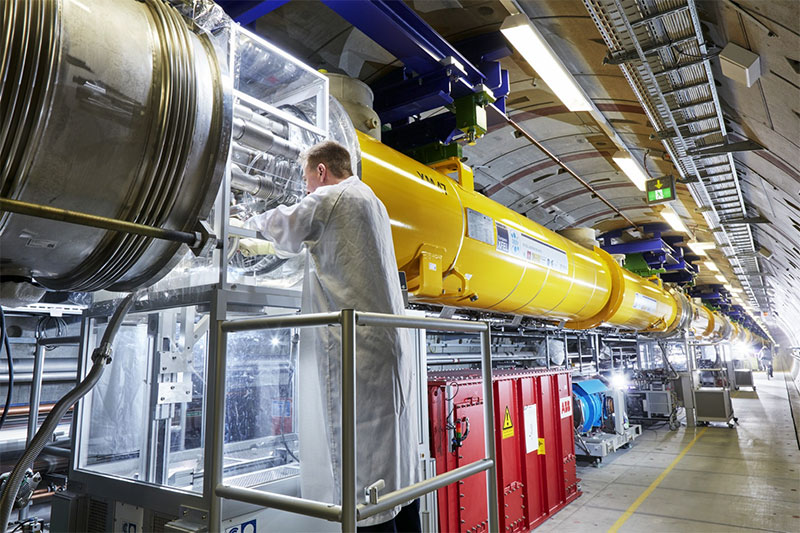
The superconducting electron accelerator, which is 1.7 kilometers long in total, consists of 96 yellow cryomodules, each 12 meters long. Special local clean room cells were developed for their setup and maintenance.
Source: European XFEL
The X-ray laser European XFEL, or short EuXFEL, is truly extraordinary: 27,000 light flashes per second at a peak luminosity of 5∙1033 Sch, more than a billion times that of conventional X-ray sources. The maximum electron energy is 17.5 GeV, about 3 GeV more than the famous LCLS accelerator in Stanford/USA. The 3.4-kilometer-long underground facility extends from the DESY campus in Hamburg to the town of Schenefeld in Schleswig-Holstein. The DESY research center is also one of EuXFEL's main shareholders, so it was possible to draw on DESY's profound particle accelerator know-how during the construction of the EuXFEL facilities.
But how can such intense X-ray light be generated? The name of the technical "trick" is SASE, short for Self-Amplified Spontaneous Emission. In this process, high-energy electron bunches are shot through an undulator, i.e. a periodic arrangement of dipole magnets, with the north-south orientation of neighboring magnets being exactly opposite in each case. This special magnet arrangement forces the electrons into a serpentine path, which subsequently makes them emit synchrotron radiation which can then be used as a focused X-ray beam for experiments.
But for the SASE process to work reliably, the electron bunches must have an enormously high peak current strength. To this end, they are generated in an injector using a high-frequency electron source and then condensed in electron pulse compressors. During the subsequent transport of the electron bunches through the kilometer-long accelerator tunnel, very special vacuum conditions must prevail so that the coherence of the electron beam – i.e. the temporally constant phase relationship of the individual electrons to each other – is not disturbed in the slightest. A constant ultrahigh vacuum must also prevail in the photon beam tubes through which the X-ray light is finally guided to the experiments.
Last but not least, an isolation vacuum system is needed for the superconducting accelerator modules and the helium supply. The high demands on the operational reliability of EuXFEL, or on the time resolution of its X-ray laser, are directly transferred to the vacuum system used.
Phil Schneider, VAT product manager for all-metal valves, puts it in a nutshell: "A good vacuum is the key to the successful operation of the entire EuXFEL X-ray laser source!"
He should know: After all, he was directly involved in the construction of the impressive facility as a vacuum engineer at the European XFEL before joining VAT. "Back then, I worked in the group in charge of the photon vacuum. Among other things, I was involved in the construction of the beamline. Today, I contribute my vacuum know-how to VAT, but I still get excited when new scientific breakthroughs are made at European XFEL."
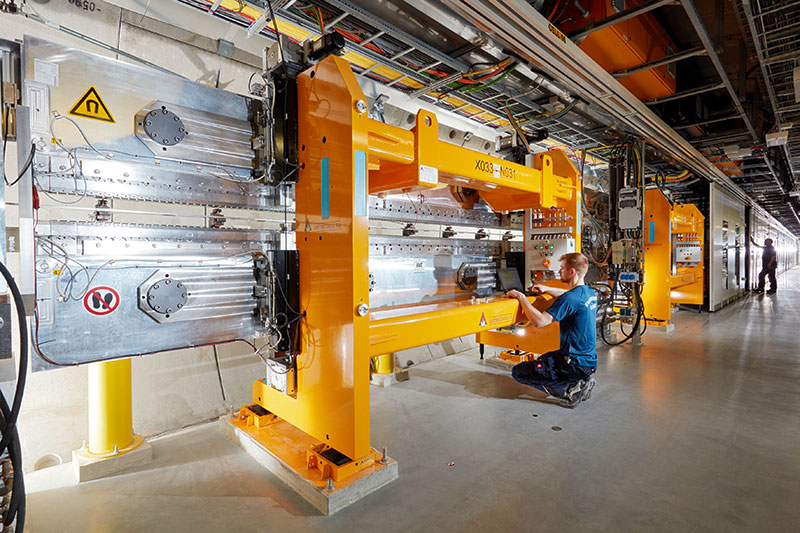
View of the undulators in the EuXFEL tunnel, where the synchrotron radiation is generated.
Source: European XFEL / Heiner Müller-Elsner
Since the various system components of the X-ray laser require highly different vacuum conditions, the EuXFEL engineers had to develop creative yet compatible vacuum concepts together with their colleagues from DESY, who are responsible for the accelerator vacuum. Thus, the electron beam vacuum was divided into different sections, including sections around the superconducting accelerator modules (operating temperature: 2 K) and sections for the rest of the accelerator vacuum (at room temperature). In addition to the total 52 sections of the accelerator system, there are another 80 sections for the photon vacuum system.
"Due to the radiation and temperature conditions prevailing at EuXFEL, the isolation of the beamlines from the experiment huts can only be guaranteed by all-metal vacuum slides in the long term. That's why all-metal vacuum slides of the VAT series 48 were installed," explains Phil Schneider. "These reliable vacuum slides are characterized by the fact that hard-on-hard sealing is possible in a repeatable manner and without plastic deformation of the sealing elements. The VATRING technology used here allows repeated, dynamic hard-on-hard closing with both sealing partners, the VATRING as well as the valve seat, being made of stainless steel."
To ensure that the electron beam in the area around the superconductors is sufficiently protected from disruptive collisions with air molecules, a pressure of no more than 10-5 mbar must prevail there. At this pressure, the electrons can be guided to the electron absorber almost without loss: Only 10 out of about one billion transported electrons stray from the path on their 2-kilometer-long flight path! On the other hand, in the accelerator sections with room temperature (for example near the electron source), the pressure must be lowered to 10-10 mbar in order to compensate for the temperature-related increased mobility of the air molecules.
Because of the extreme conditions, all-metal valves are necessary for most EuXFEL applications. But there are also areas within the facility where the valve load in terms of temperature, radiation, and vacuum level is sufficiently low to consider the use of valves with elastomer seals. These have the advantage of higher cycle values, thus minimizing the amount of valve maintenance required.
"For example, elastomer-sealed UHV gate valves of the VAT series 01.0, in size DN40, were installed near the undulators," recalls Phil Schneider. "And elastomer-sealed gate valves also proved to be the optimal solution for separating the individual sector areas for maintenance purposes."
With a total length of 3.4 km, the EuXFEL facility placed exceptionally high demands on the developers and suppliers. "EuXFEL's cable requirements alone are enormous!" recalls Felix Jordan, the Sales Manager responsible at VAT.
Since the vacuum systems also extend over very long distances and large volumes, sudden air infiltrations pose a very special challenge. It is easy to imagine the huge amount of time – and thus costs – that would be involved in just restoring the required vacuum level! To reliably protect the operation of the beam transport vacuum system against such air inrushes, a safety system based on quick-closing valves of the VAT series 75 (in sizes DN40 and DN100) ensures that the vacuum system is automatically shut off within fractions of a second in the event of an unforeseen pressure increase in one of the adjacent sectors.
Phil Schneider explains: "With their enormously fast closing times, for example 15 ms for the DN100 valve, the VAT series 75 valves provide very immediate protection for the concerned parts of the system. In the event of a leak, all relevant sector valves are also closed, of course. But thanks to the quick-closing valves, their longer closing times are of no consequence."
To vent the beamline with nitrogen and to pump it out with mobile pumping stations, the EuXFEL developers have installed UHV all-metal angle valves of the VAT series 54.1, in size DN16. The venting valve is equipped with a special particle filter to protect the highly sensitive X-ray mirror surfaces from contamination by incoming particles. There is also an increased risk of contamination when pumping out and venting the electron beam vacuum, partly due to turbulence.
"In the first setups, we found that particles the size of micrometers may be carried along by a flow that is too turbulent," Phil Schneider recalls of the technical challenge. Here, too, the reliable controllability of the VAT series 54.1 valves now ensures a laminar gas flow during the pump-down and venting cycles.